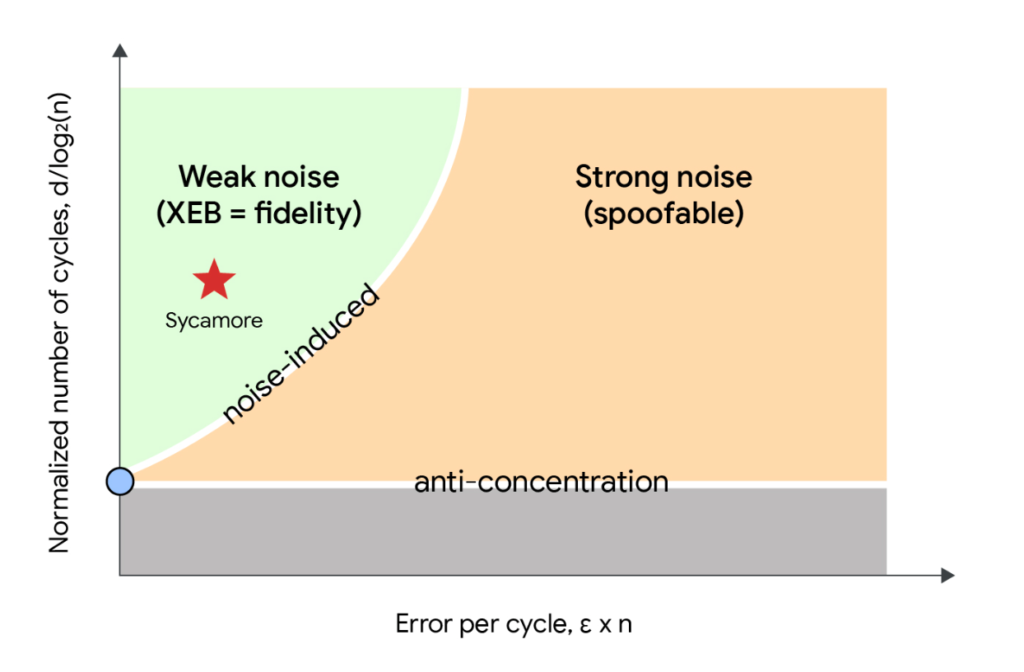
Google Quantum AI has achieved a breakthrough by doubling the quantum circuit volume in its Random Circuit Sampling (RCS) benchmark. This accomplishment was realized using the Sycamore processor, where the complexity of quantum computations was increased while maintaining the same fidelity as the benchmarks established in 2019. The updated RCS benchmark continues to serve as a useful tool for evaluating the performance of quantum computers in tasks that remain challenging for classical supercomputers to simulate.
In the recent experiment, the Sycamore processor successfully executed circuits comprising 67 qubits and 32 cycles, involving a total of 880 two-qubit gates. This effectively enhances the circuit volume compared to previous results while preserving a fidelity measure of 1.5 × 10⁻³. This outcome indicates that Google’s quantum systems can manage more intricate circuits in the presence of noise, a common issue that tends to compromise quantum computation integrity.
Noise accumulation during quantum computations presents ongoing challenges, and Google’s research identifies two distinct phase transitions that occur as noise levels increase alongside the number of qubits. These transitions reveal the operational behavior of quantum systems under varying noise conditions. Notably, the results indicate that the Sycamore processor operates within a low-noise regime, which is crucial for enabling quantum advantage—where quantum systems can outperform classical systems.
To validate the fidelity of the RCS results, the research team employed cross-entropy benchmarking (XEB). This method involves a comparison between the outputs of a noisy quantum processor and those of an ideal, noise-free system. By dividing the quantum processor into smaller segments, researchers were able to estimate the overall fidelity, which remained stable despite the increase in circuit volume. This reinforces the reliability of RCS as a benchmarking approach, even under noisy conditions.
Lastly, the study examined the efficacy of classical “spoofing” algorithms that attempt to mimic quantum performance in RCS scenarios. The results revealed that such classical methods were ineffective in replicating the outcomes produced by Google’s quantum processor. This finding further supports the notion that RCS is a credible means of assessing the capabilities of quantum hardware as the systems scale in size and complexity, ultimately emphasizing the distinct advantages of quantum computing over classical approaches in specific applications.
For more details, visit Google Quantum AI’s blog here or read the full paper in Nature here.
October 12, 2024