By Michael Baczyk
By weaving together scenarios analysis, technological roadmapping, and quantitative market modeling, GQI empowers decision-makers to navigate the uncertainties inherent to quantum – yes, pun intended.
Now, let me walk you through how GQI accomplishes this exactly.
Scenarios analysis
By developing a range of potential futures (Figure 1) – from optimistic to pessimistic, bull to bear – investors and stakeholders can better prepare for various outcomes and make more informed decisions.
GQI’s scenario framework spans across quantum computing, communications, and sensing, recognizing the interconnected nature of these technologies. In quantum computing, scenarios range from “Broad NISQ Quantum Advantage” where near-term devices deliver commercial value, to “Hard Quantum Winter” where progress stalls, to various paths towards fault-tolerant quantum computing (FTQC). Quantum communications scenarios address the evolution of quantum-safe cryptography and the development of quantum networks. Quantum sensing scenarios explore the progression from niche applications to widespread dual-use technologies.
Consider a quantum computing full-stack provider. These scenarios inform decisions on resource allocation across hardware, software, and applications development. A “Broad NISQ Quantum Advantage” scenario might prioritize near-term application development and customer engagements, while preparation for “Eye of the Needle FTQC” would emphasize fundamental research into error correction. While, hardware-focused quantum computing vendors must consider scenarios like “Distributed FTQC” versus “Goliath FTQC” when planning their scaling strategies.
Scenarios descriptions for reference:
- Broad NISQ Quantum Advantage: Near-term quantum devices deliver commercial value across diverse applications.
- Hard Quantum Winter: Progress stalls, commercial applications struggle to materialize.
- Broad Early FTQC: Multiple players achieve small-scale fault-tolerant quantum computing.
- Eye of the Needle FTQC: Only the most robust approaches succeed in delivering practical FTQC.
- Distributed FTQC: Large-scale quantum computing achieved through networked smaller modules.
- Goliath FTQC: Very large, centralized systems required for meaningful quantum advantage.
- Turbo FTQC: Dramatically improved FTQC enables even algorithms with modest speedups to become practical.
- Quantum Safe Migration: Widespread adoption of post-quantum cryptography.
- Quantum Resistant Networks: Development of communication networks resilient to quantum attacks.
- Quantum Enabled Networks: Quantum technologies enhance network capabilities and security.
- Quantum Internets: Fully realized quantum communication networks enabling distributed quantum computing.
- Mission Based QS: Quantum sensing adopted for specialized, high-value applications.
- Accelerated Military QS Adoption: Rapid integration of quantum sensors in defense applications.
- Dual-Use QS Evolution: Quantum sensing technologies find widespread commercial and military use.
Technological roadmapping
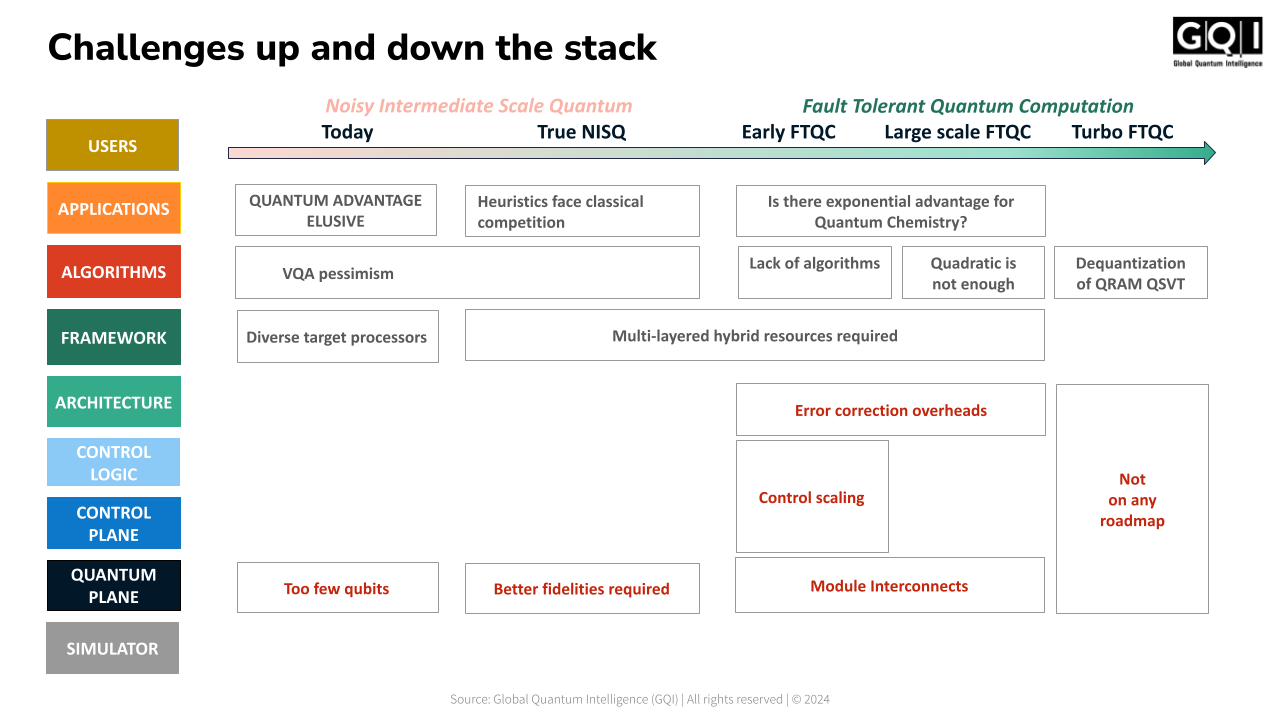
The trajectory of quantum technology development, whether optimistic or pessimistic, hinges on the industry’s ability to overcome challenges present throughout the entire quantum stack – the illustration for Quantum Computing provided in Figure 2.
At the quantum plane level, current systems grapple with having too few qubits and insufficient fidelities. As we move towards Early FTQC, implementation of module interconnects become critical. The control plane and control logic face scaling challenges as systems grow more complex. In the architecture layer, error correction overheads emerge as a significant hurdle for FTQC. The framework layer consistently requires multi-layered hybrid resources across all stages of development. At the algorithmic level, we see a progression from current VQA (Variational Quantum Algorithm) pessimism to concerns about the lack of algorithms for specific applications in the FTQC era, and eventually to questions about the sufficiency of quadratic speedups and the potential dequantization of certain algorithms.
GQI’s approach to assessing technological progress, as shown in the Figure 3, maps these challenges to specific eras and quantifiable metrics. The framework tracks the relationship between physical error rates, quantum error correction codes, and the resulting logical error rates. This progression is divided into distinct eras – Intermediate, Early, Large Scale, and Mature – each characterized by increasing qubit counts and decreasing error rates. For instance, the Intermediate era (KiloQuop) requires logical error rates around 10-3 to 10-5, while the Mature era (PetaQuop) demands logical error rates as low as 10-12 to 10-16.
These technical regimes are then mapped to commercial scenarios, providing a comprehensive view of how technological advancements translate into market opportunities. The Broad NISQ Quantum Advantage scenario, for example, aligns with the Intermediate era, requiring modest logical qubit counts but still presenting significant challenges in achieving quantum advantage. In contrast, the Goliath FTQC scenario corresponds to the Mature era, demanding extreme levels of qubit performance and error correction to enable large-scale quantum computations.
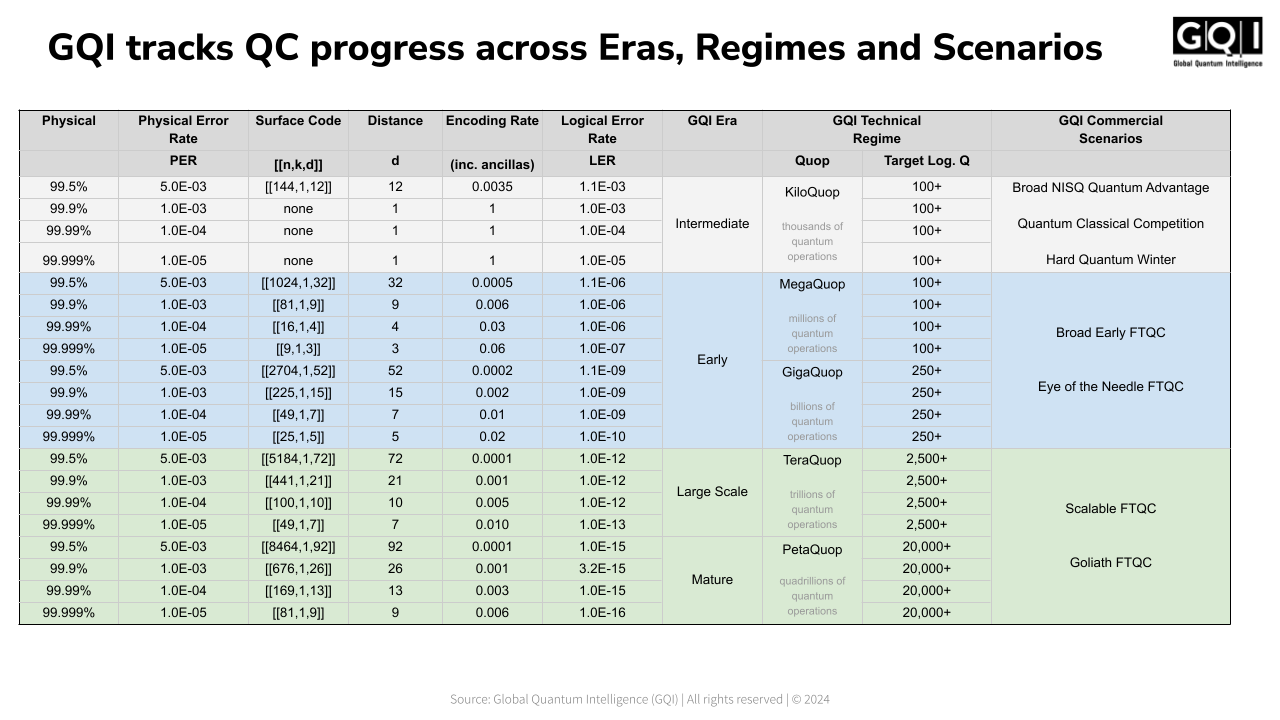
Quantitative QAM model
GQI’s Quantum Addressable Market (QAM) model synthesizes scenario analysis, technological milestones, and market dynamics to provide a quantitative assessment of the quantum technology landscape. The QAM model’s power lies in its ability to link technological progress to market potential across various scenarios. As illustrated in Figure 4, which covers the period from 2024 to 2045, we see the potential market size evolving from a mere few billion dollars to over $600 billion. This growth is not uniform across all quantum technology sectors but is driven by the interplay of different scenarios unfolding over time.
In the near term, as shown in the second image focusing on 2024-2030, we observe the dominance of Quantum Safe Migration, reflecting the urgent need for cybersecurity measures in the face of advancing quantum capabilities. Concurrently, we see the gradual emergence of Quantum Pioneers and Broad NISQ Quantum Advantage scenarios, indicating the initial commercialization of quantum technologies.
As we move into the medium and long term, the model projects the rise of more advanced scenarios such as Distributed FTQC, Goliath FTQC, and Quantum Internets. These scenarios represent the realization of large-scale quantum computing and quantum communication networks, unlocking umatched computational power and secure communication capabilities.
Crucially, the QAM model also allows for flexible analysis across different market segments and value chain positions. For instance for a midstack provider, GQI could use this model to assess their addressable market within specific scenarios and timeframes. By overlaying the technological progress required for each scenario with the provider’s capabilities and roadmap, we can derive a nuanced understanding of their market opportunity. This is particularly valuable in due diligence processes, where understanding the specific addressable market for a company’s offering is crucial.
Closing words
We’ve explored how scenario analysis intertwines with technological milestones, and how these translate into quantifiable market opportunities.
Whether you’re an investor looking to make informed decisions in the quantum sector, or a startup seeking to articulate your value proposition in this complex landscape, we invite you to reach out. Contact us at info@global-qi.com. After all, in the world of quantum technologies, the future isn’t just uncertain – it’s entangled with possibilities.
August 28, 2024